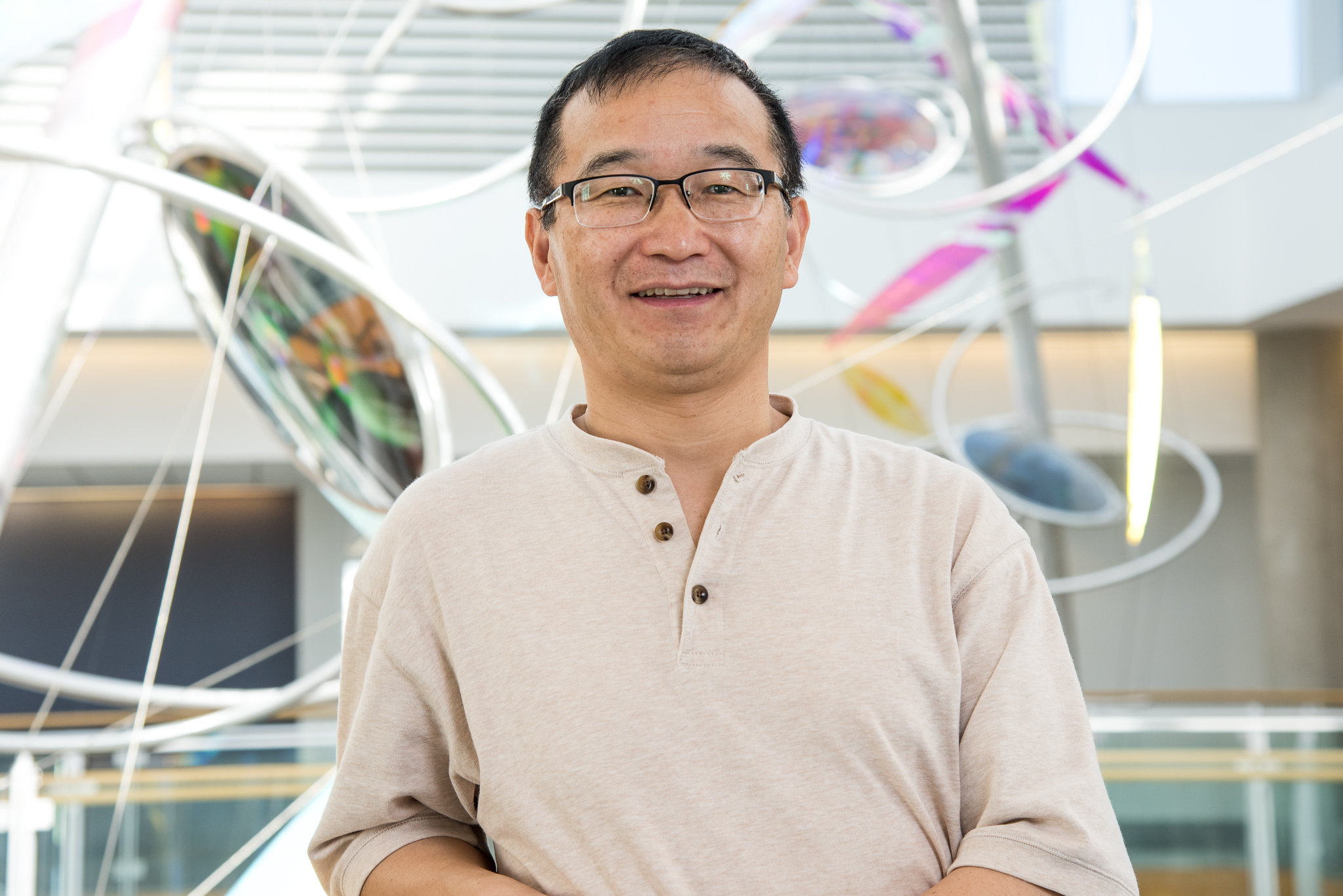
ST. LOUIS, MO – The Academy of Science – St. Louis is proud to announce the recipients of the 27th Annual Outstanding St. Louis Scientists Awards, recognizing individuals and organizations that have made significant contributions to the advancement of science, engineering, and technology. The awards ceremony will be held on April 3, 2025, at the Missouri Botanical Garden.
Since its inception, the Outstanding St. Louis Scientists Awards has honored some of the brightest minds in the region, celebrating their exceptional achievements, groundbreaking discoveries, and lasting impact on science and society. This year’s honorees represent a diverse array of disciplines, from plant science and medicine to artificial intelligence and STEM education.
2025 Award Recipients
Fellows Award (Outstanding Achievement in Science)
● Dr. Steven Levine, Bayer – A global leader in ecotoxicology, Dr. Levine has made pioneering contributions to environmental safety assessments for crop protection.
● Dr. Ram Dixit, Washington University – A distinguished biologist whose research on the cytoskeleton is enhancing our understanding of cell shape and plant morphogenesis.
George Engelmann Interdisciplinary Award (Collaborative Science Achievement)
● Dr. Allison Miller, Donald Danforth Plant Science Center & St. Louis University – An innovative leader in plant science, engaged in interdisciplinary approaches to explore biology, evolution and root-shoot interactions to support sustainable agriculture systems.
Innovation Award (Exceptional Potential in Science, Engineering, or Technology)
● Dr. Peng Bai, Washington University in St. Louis – A trailblazer in battery research, developing new methods to improve energy storage and efficiency.
● Dr. Phani Chavali, Bayer – A machine learning and AI expert revolutionizing plant breeding to accelerate crop development and genetic gain.James B. Eads Award (Excellence in Engineering or Technology)
● Dr. Bing Yang, University of Missouri & Donald Danforth Plant Science Center – A world-renowned researcher in plant genome engineering, advancing CRISPR/Cas technology for disease-resistant crops.
Peter H. Raven Lifetime Achievement Award (Career of Service in Science, Engineering, or Technology)
● Mary E. Burke, MA, AAAS Fellow, Retired CEO, The Academy of Science – St. Louis – A transformative leader in STEM education and outreach, expanding science accessibility across the region.
Science Educator Award (Excellence in Science Education)
● Dr. Farzana Hoque, St. Louis University – A dedicated medical educator and mentor, advancing diversity, equity, and health awareness in the medical sciences.
● Peggy James Nacke, Retired Director of Special Projects and Events, The Academy of Science – St. Louis
– A champion of citizen science, spearheading groundbreaking STEM initiatives like the region’s first e-Science Fair and BioBlitz programs.
Science Leadership Award (Leadership in Advancing Science and Scientists)
● Dr. Thomas Eickhoff, Bayer – A mentor and industry leader driving innovation in agriculture and digital farming.
● MilliporeSigma, the Life Science business of Merck KGaA, Darmstadt, Germany – A St. Louis-based global leader in life sciences and pharmaceutical development, committed to STEM education and workforce development.
Deborah Patterson Award (Broadening Participation in STEM – Inaugural Award)
● Deborah Patterson, Former President of the Monsanto Fund – A tireless advocate for inclusive STEM education, instrumental in establishing STEMpact and STEMSTL.
A Celebration of Science and Innovation
The Outstanding St. Louis Scientists Awards Ceremony will bring together the region’s scientific, academic, and business communities to celebrate the honorees’ remarkable achievements. The event will also highlight the Academy’s ongoing commitment to fostering science literacy and inspiring the next generation of innovators.
For more information about the event, sponsorship opportunities, or to purchase tickets, please visit
www.academyofsciencestl.org or contact Executive Director Kate Polokonis (kpolokonis@academyofsciencestl.org).
About The Academy of Science – St. Louis Founded in 1856, The Academy of Science – St. Louis is a non-profit organization dedicated to promoting science literacy, education, and collaboration throughout the region. Through public seminars, student programs, and community engagement, the Academy continues to inspire scientific curiosity and discovery.