Mizzou researchers genetically engineer plants to optimize microscopy
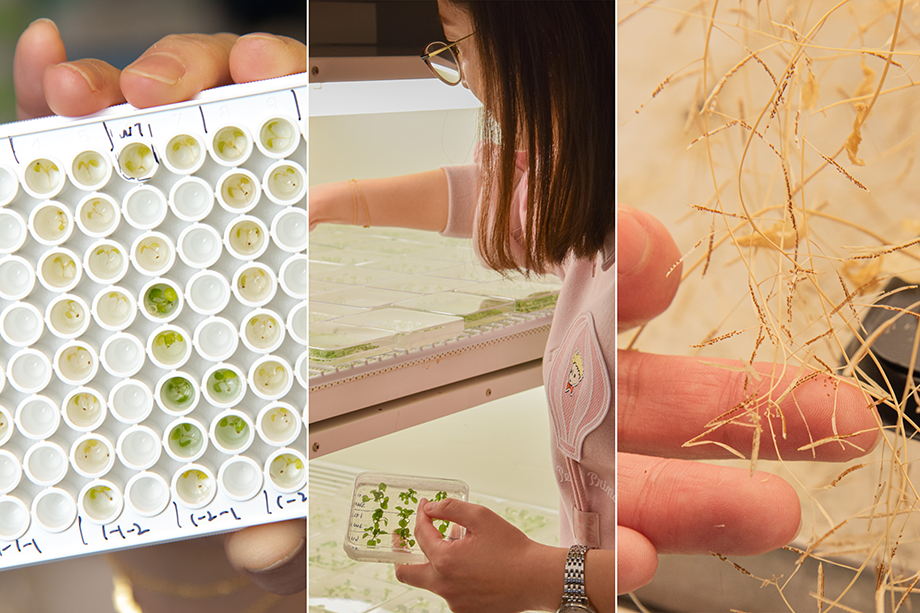
Gary Stacey’s lab is a breeding ground for model plants, curated to get the most precise image of the plant leaf tissues.
Those plants help scientists at Mizzou and the Environmental Molecular Sciences Laboratory (EMSL) bridge plant science and microscopy to capture high quality snapshots of a plant’s cellular structure. The collaborators recently co-published their latest contributions to lattice light-sheet microscopy imaging in The Biophysical Journal. It shows a way to better see plant plasma membrane-localized receptors that previously were obscured by the background glow of chlorophyll-like objects.
A plant that’s easier to see (under the microscope)
Mengran Yang’s life’s work is studying cellular proteins in plants, so she plays a big part in this partnership. Since she joined the Stacey lab in Bond Life Sciences at Mizzou as a postdoctoral fellow, Yang has immersed herself in the cellular components of models like Arabidopsis, a small mustard plant used ubiquitously in plant research. Whenever people know about Arabidopsis, Yang gleams with excitement.
“We want to use microscopic technologies to solve biology questions,” Yang said about the reason the collaboration came to be.
This collaboration with EMSL – a DOE Scientific User Facility located at the Pacific Northwest National Laboratory – will create precision imaging that can help future researchers understand subcellular location of proteins that contribute to a crop’s disease resistance or optimize farming to yield the best harvest, among other applications.
Yang has never visited Washington nor met the researchers from EMSL in person, but despite the distance she said she has vested trust in the team to share high resolution results.
Yang curates a handful of genetically modified or transgenic plants which produce thousands of seeds. She then sends the seeds off to a laboratory in Washington, where her collaborators grow their own plants optimized for light-sheet microscopy. Without this genetic modification, EMSL wouldn’t be able to analyze cellular components of plants with great detail let alone fulfill their quest to filter out chlorophyll.
Yang stashes the seeds of the genetically manufactured plants in Petri dishes where they emerge in their green leafy glory roughly 10 days later. She plants the newly sprouted seedlings that grow tall, decorated with white flowers. Afterwards, she tests the phenotype of the plants to ensure they are truly genetically modified. This process could take months.
She leaves the plants to shrivel, making it prime time to gather seeds. Yang is there for every stage of the plant’s life cycle, and she even sees them off as they are shipped across the country.
“Conducting this process with transgenic plants is very time consuming from phenotyping to harvesting,” Yang said. After seeing the images from EMSLs microscope, Yang added it makes her feel like her work is worth it.
One of the beauties of studying plants is because they “have more receptors localized on the plasma membrane compared to animals,” Yang said. This very factor lured her into studying Arabidopsis.
From modified plant to a clearer picture
The Stacey lab has spent more than a decade working with EMSL, because they need each other. This work wouldn’t be possible without the Stacey lab’s sharing its plant engineering know-how and EMSL contributing its national laboratory imaging prowess.
“Humans are social animals,” Stacey said about the natural urge to collaborate in the scientific research realm.
The researchers enhanced clarity in their pictures by getting around natural plant pigments that cloud their results. Chlorophyll — the compound that both gives the plant its color and is responsible for absorbing energy from the sun — is a nuisance for florescence microscopy imaging. It pollutes an image with background light and compounds the challenge the plant cell wall presents, which makes it difficult to tag internal cell parts with fluorescent markers needed to see plant receptors and other cell parts under a microscope.
Yang genetically engineers DNA that is inserted into the plant cell, making the receptors of the plant have the ideal functions for fluorescent viewing. The receptors are fused to a tag, thanks to this genetic process, which faces the outside of the cell making it easier for antibodies to bind to them. These antibodies are ready for fluorescent viewing. These tags glow when excited with a certain wavelength of light, making them stand out from other cell structures.
This method is the first of its kind, letting scientists see the location of a protein at a molecular resolution.
Because Yang’s research addresses fundamental questions in plant biology, she said it may appear “useless” to those not immersed in the field. But, seeing cell parts is a vital step to understanding how they contribute to things like plant defense. She joked that when she explains her research to her parents, she is met with looks of confusion.
“Progress (that impact humankind) is based on thousands or millions of basic research findings,” she said.
The study “Lattice light-sheet microscopy allows for super-resolution imaging of receptors in leaf tissue” published in the Biophysical Journal in February 2025. This research is funded by grants from the U.S. Department of Energy Biological and Environmental Research (DOE-BER), the National Science Foundation and the National Institute of General Medical Sciences of the National Institutes of Health.