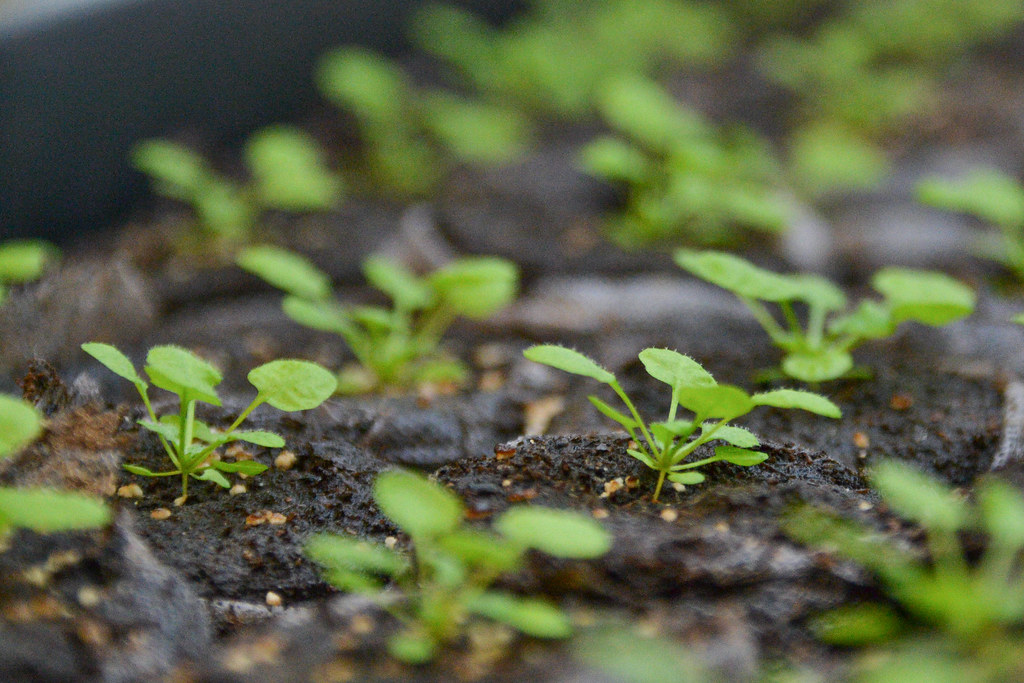
By Lauren Hines | Bond LSC
Daylight might not seem dangerous, but for plants, too much daylight can cause hazards similar to a nasty sunburn or a human scalding themselves.
When you jerk your hand back from a boiling pan or a hot faucet, in a millisecond electrical signals are sent up your arm to tell your brain to move away. Like this reaction, plants have their own protective reactions to too much light.
“I think what you need to understand is that plants can’t survive without light,” said Ron Mittler, principal investigator at the Bond Life Sciences Center. “Light is the master of the plant basically.”
Mittler’s lab discovered that light receptor phytochrome B is responsible for triggering the signals for light danger that reverberate from one cell to another and not chloroplasts as previously thought. Understanding how plants respond can help build a picture of a process called systemic signaling to help researchers create genetically modified crops to withstand these stresses. Their results were recently published in the Plant Physiology journal.
The scientific community thought if light was put on a plant, the organelles that conduct photosynthesis, called chloroplasts, would trigger systemic signaling and the ROS wave.
“It’s really about having this spreadsheet of knowledge and not knowing where the pieces of the puzzle from high light-reactive action to chloroplast or to stomatal closure, where all those pieces fit,” said Mannie Liscum, collaborator and Mizzou professor of biological sciences.
The ROS wave, named by Mittler in 2009, is the process where reactive oxygen triggers a cell reaction that subsequently triggers the cell next to it and so on creating a wave of activation. Reactive oxygen is a tricky molecule that reacts very easily. It can cause destruction in cells with these reactions or use its reactivity to send a message in a proactive way between many cells.
“What we found is that if we use a phytochrome mutant — phytochrome B in particular — we completely eliminate this ROS production by high light stress,” Mittler said. “To me, it was really amazing because everybody else thought that the light would impact the chloroplast.”
While chloroplasts absorb light to turn into energy, phytochromes can take certain types of light and turn genes on. Depending on the type of phytochrome, they can take in different wavelengths of light and help plants understand light intensity and light quality. In turn, this helps a leaf or a stem figure out where to grow and if light is coming through shade.
The Mittler and Liscum labs used the sensitivity of the light sensor to their advantage.
“By using different wavelengths, different colors of light, we started narrowing down which of the sensory systems in the plant were actually doing the job,” Liscum said.
Eventually, they found responses to red light. This meant that phytochromes, especially phytochrome B, was responsible.
However, just like how we all learn to not touch the hot stove after our first burn, researchers also found that the ROS wave triggered a systemic stress memory mechanism. That meant the plant became familiar with the stress and its response was stronger.
“Evolution has realized that these high light intensities don’t just show up and instantaneously leave, but that they might stay around for a while,” Liscum said. “Evolution is adapted to have some system for the chemistry in the plant to change for some period of time before it goes back to what it was before it saw the stress.”
While the paper revealed much about phytochrome B and the systemic response to light stress, the Mittler and Liscum labs want to further investigate the memory and mechanistic aspects of the process.
“The next stage is to figure out how the sensing of the light stress by the receptors and not by the chloroplast triggers this response,” Mittler said.