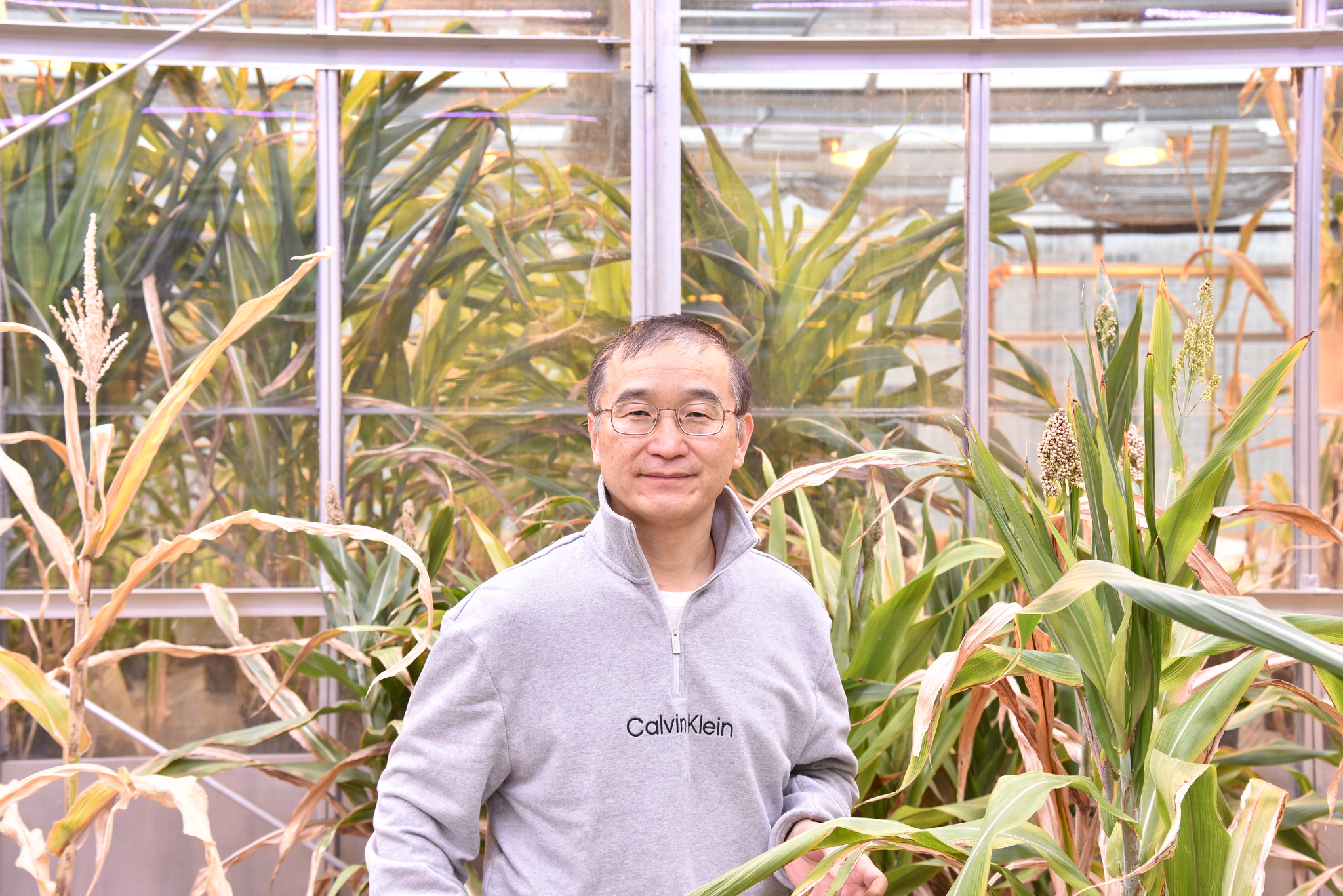
By Josie Heimsoth | Bond LSC
When it comes to making better crops, CRISPR-Cas9 based gene editing have revolutionized plant science with its ability to more precisely and quickly alter plant DNA.
But the technology can also aid researchers in finding weaknesses in the enemies of crops like rice.
Bing Yang, a Bond Life Sciences Center researcher and MU professor of plant sciences, recently used the genome editing tool to modify bacteria responsible for rice blight, and it may be the answer scientists are looking for. He recently published his results in the journal Nature Communications Biology.
Bacterial blight causes a tremendous reduction in rice yields, and researchers, including Yang, made it their goal to understand the genetic basis of the bacteria to provide insight into host-pathogen interactions to plan effective control of blight.
“This disease could be a model system for the study of bacterial pathogens to effectively understand more crop diseases,” Yang said. “If we understand the disease, it can help us reduce other chronic diseases.”
Bacterial blight also causes the wilting and yellowing of leaves. Plants battle the microscopic bacteria, which colonize inside tissues to prevent the plant’s ability to deliver water and nutrients to the rest of the plant. These invasive bacteria do this to achieve their only purpose, which is to survive and reproduce.
The bacteria tend to thrive when the plant is at its weakest, “so that’s why there’s no effective and chemical way to cure or to control the disease,” Yang said. “The only effective way is making it genetically resistant.”
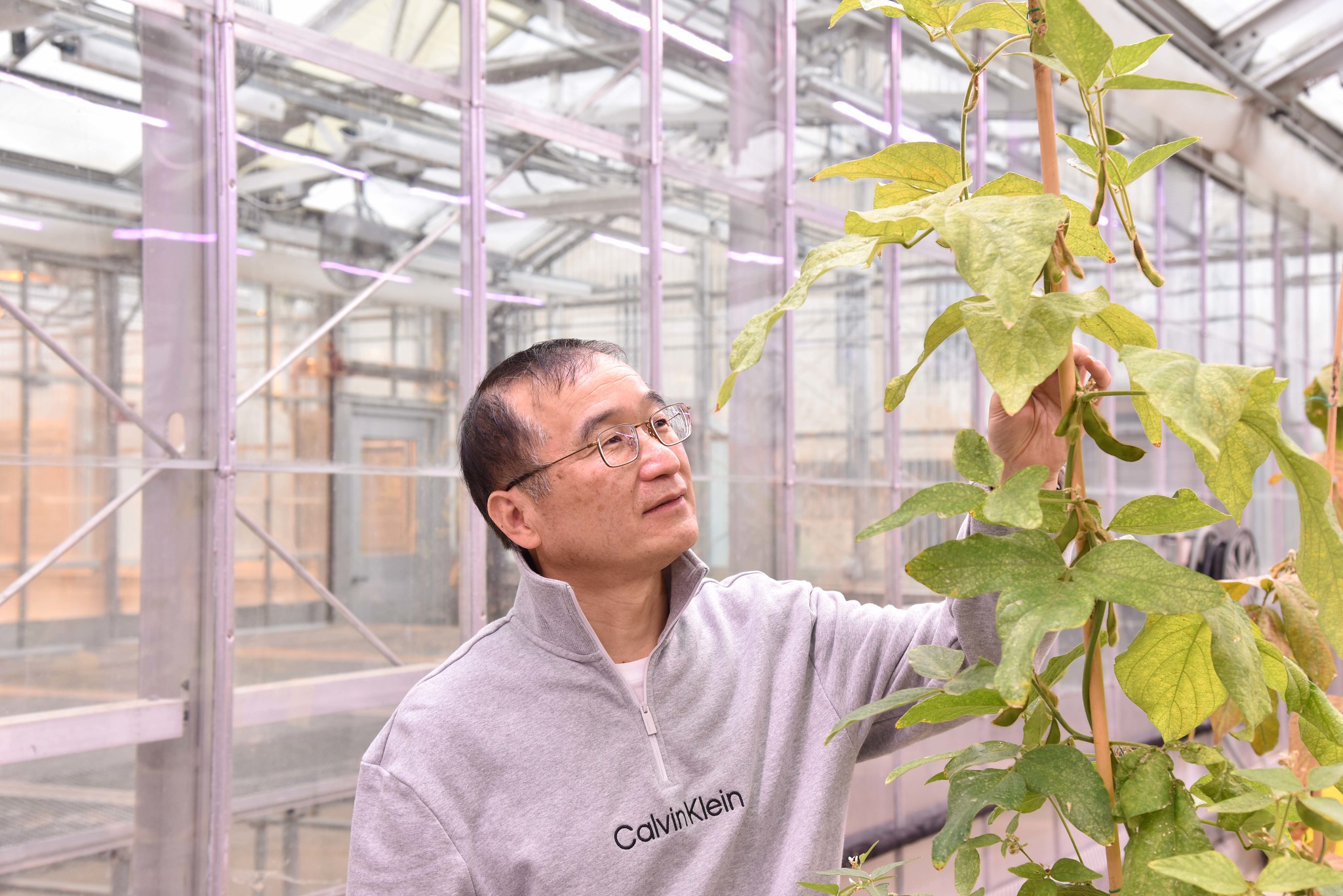
Though CRISPR-Cas9 can modify plants by precisely cutting DNA and then letting natural DNA repairing processes take over, bacteria genes can’t be edited that easily.
When infecting plants, bacteria try to take over the sucrose transporter system, which transports sugar out of resource and engages in plant growth and responses to abiotic stress. Without editing bacteria, there is no way of telling which genes play a role in causing disease, so Yang’s lab modified different genes with CRISPR-Cas9 deaminase until a modified bacteria stopped hijacking a plant and killing it.
“If that [edited] gene in the bacteria cannot cause disease in the rice, it means that gene plays an essential role to help the bacteria cause disease,” Yang said.
CRISPR-Cas9 deaminase is what Yang’s lab uses for a wide range of genome editing applications in eukaryotes and prokaryotes. To achieve the result they desired, they needed to produce the right amount of CRISPR-Cas9 deaminase, otherwise it cannot have a desired effect on the bacteria cells.
“You want to express CRISPR-Cas9 deaminase in the bacteria cell, but not make it too high or too low,” Yang said. “If the deaminase is too low, you won’t see the effect on the bacteria, but if it’s too high, it kills the bacteria cell. You need to find the good sum.”
For Yang, rice is a crop that has been in his life from childhood in China to his current research. His father farmed the crop, and now, Yang continues to study its most significant disease, which is capable of killing up to 75 percent of a rice crop.
One of the benefits that sets CRISPR-Cas9 technology apart from others is it has given Yang’s lab results faster. Bacterial gene editing takes time and usually other methods have taken several months, while CRISPR-Cas9 deaminase gave them results in a number of weeks, making it a speedy research tool.
“You can look into the bacterial genes in groups and see which group helps the bacteria infect the rice,” Yang said. “It tremendously saves time and unlocks the bacteria much easier.”
These efforts are changing the game for gene modifying in bacteria, and with time could stop bacteria from having devastating impacts. Not only will the research help in rice, but can also serve with understanding bacterial disease in other plants, too.
The next step is to make this gene editing system more efficient.
“This is a very useful tool, which is not only benefited my lab, but also other labs which work on plant diseases,” Yang said.
Yang’s research is published in the paper, “Efficient CRISPR-Cas9 based cytosine base editors for phytopathogenic bacteria” in the publication Nature Communications Biology on January 17, 2023. You can read more about the study here.